The sustainability and reliability of structures is of central importance for mobility and regenerative energy from a technical and economic point of view. Accordingly, understanding the structural and material behavior under different environmental influences (temperature, humidity, etc.) and the ability to predict damage play a key role in the development of lightweight components, reliable systems and the achievement of low electricity generation costs. Physically motivated numerical simulation models are an essential part of the development process of sustainable structures. In order to increase the efficiency of the simulation models and thus expand the area of application, reduction methods and machine learning are used. The Composites department combines the concepts of sustainability, service life and method efficiency. The scientific work is divided into the following main research areas:
Composite Materials
New and detailed simulation models are developed in the Composite Materials group. Material laws and failure models of fiber composites are developed here on different scales from the nano to the meso scale. At the nano-level, atomistic simulation methods are used to model the atomic components by using the molecular dynamics, while at the micro- and meso-level physically motivated constitutive material models are developed and integrated in a finite element framework. The material models take into account non-linear viscoelasticities, viscoplasticities, damage and various environmental influences. In addition, the Materials group is increasingly relying on machine learning, which improves our material models and at the same time leads to an increase of efficiency.
Composite Structures
This team is concerned with the stability analysis and dynamic analysis of slender and thin-walled structures. A second theme is the fatigue analysis of composite structures. Important aspects in this team are probabilistic analysis and reduced order modeling.
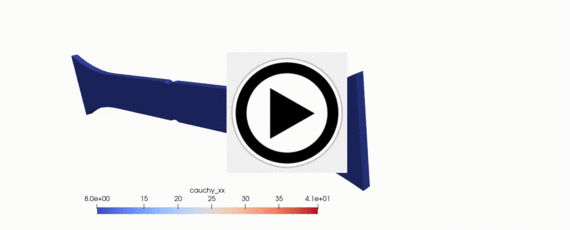

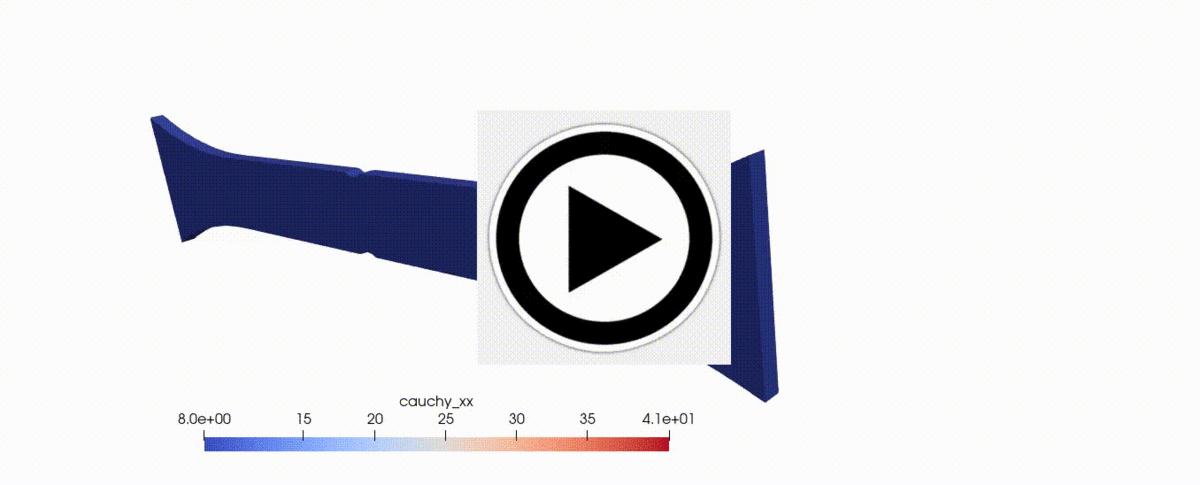
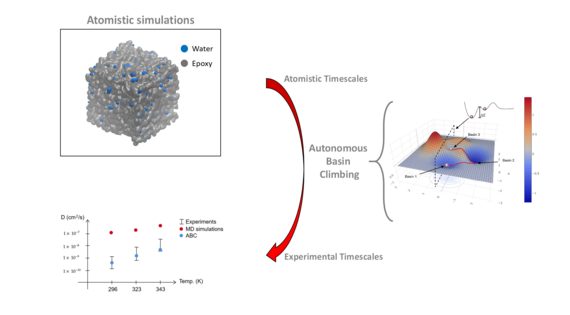
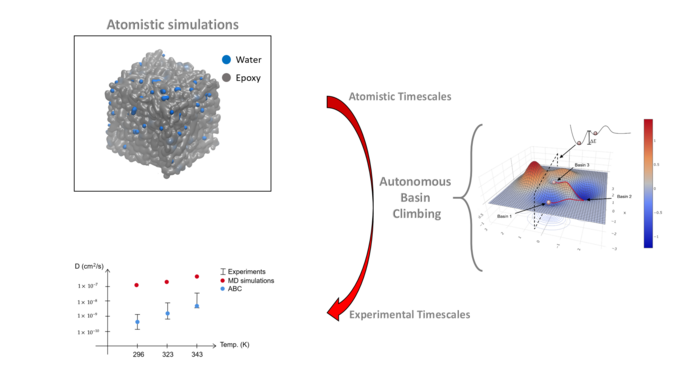
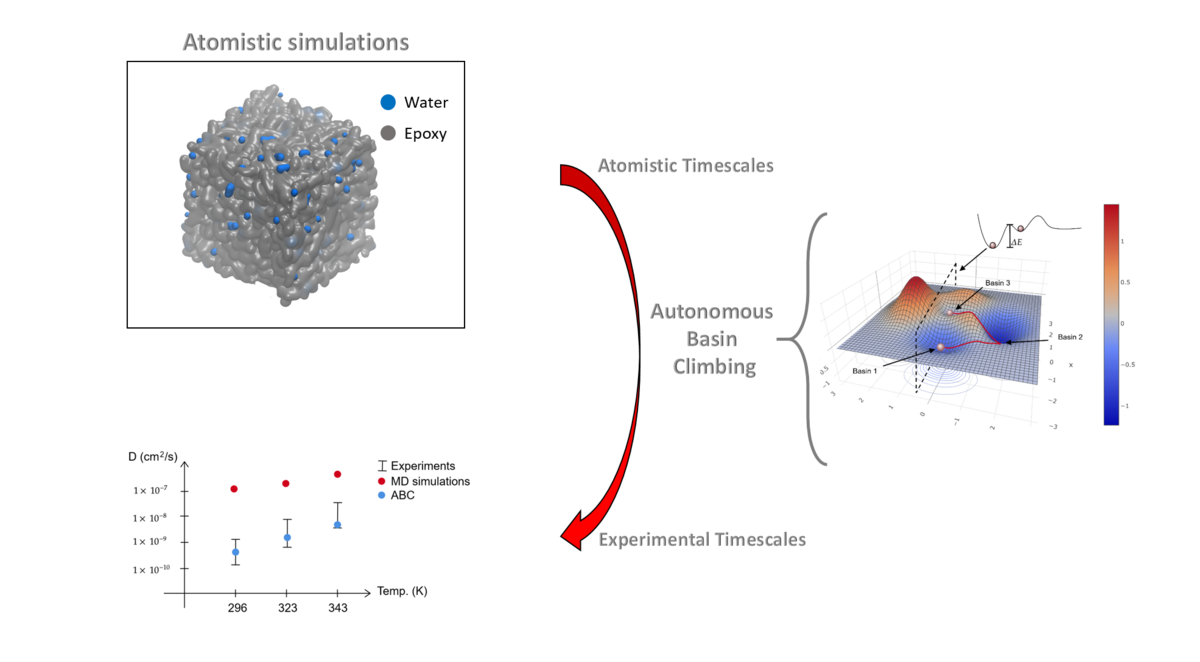
Recent research projects
Material Modeling
-
Virtual Materials and their Validation: German-French School of Computational Engineering (ViVaCE)Compressive failure mechanism of unidirectional fibre composites has been studied extensively over the past decades. Stochastic fibre misalignments were identified as an essential factor in the prediction of compressive strength. There is a need to characterize the effects of distribution of misalignment on the strength values in compressive regime. Hence, the scope of this project is to further the development in this regard and extend the definition of failure surfaces under compressively dominated loads by statistical information. A probabilistic definition of failure surface based on imperfections at micro level, and a subsequent experimental validation are the goals of the project. This would lead to subsequent better representation of material properties at the macro scale.Led by: Prof. Dr-Ing. habil. Raimund RolfesTeam:Year: 2016Funding: Deutsche Forschungsgemeinschaft – DFG (International Research and Training Group IRTG1627)Duration: 01.12.2016 – 30.09.2019
-
Development and validation of a virtual process chain for composite structural components considering imperfections with application to a rotor blade component (Prosim R)Within the scope of this research project, the essential parts of the process chain in the production of a rotor blade are to be numerically simulated and fundamentally investigated. The primary goal is the reduction of defects in the production of fiber composite materials with the help of simulating the full process chain (manufacturing simulation and structural analysis). In order to obtain a statement on material behaviour and progressive failure, the ISD will extend the sequential multi-scale analysis by including imperfections at the ISD. The results of the draping and infusion simulation are thereby the input information.Led by: Prof. Dr-Ing. habil. Raimund RolfesTeam:Year: 2017Funding: German Research Foundation (DFG) - Project number 329147126Duration: 01.08.2017 – 31.07.2020
-
Challenges of industrial application of nanomodified and hybrid material systems in lightweight rotor blade design (HANNAH)The HANNAH research project is the follow-up to the LENAH research project. In LENAH, material systems from the fields of nanomodified materials and hybrid laminates were developed, tested and numerically simulated. This allowed the high potential of these material systems for the application in rotor blade design to be demonstrated under laboratory conditions. The investigated material systems are far superior to currently established materials, especially with regard to fatigue resistance. In the follow-up project HANNAH the (further) development of production and simulation methods for these material systems for industrial standards is now in the foreground. On the one hand, the aim is to guarantee the excellent properties of the developed material systems in large-scale production and to be able to simulate the mechanical behaviour to answer industry-related issues. In this context, the ISD develops material-specific simulation models in order increase time and cost efficiency for processes of material development and component design for nanomodified materials and hybrid laminates.Led by: Prof. Dr-Ing. habil. Raimund RolfesTeam:Year: 2019Funding: Bundesministerium für Wirtschaft und Energie (BMWI) - FKZ 0324345ADuration: 01.03.2019 – 28.02.2022
-
Modeling and simulation of the fatigue damage behavior of fiber composites under variable block loading conditionsThe goal of this research project is the extension and application of a progressive fatigue damage model for unidirectional multi-layered fiber composites for damage analysis under variable cyclic block loading patterns. The focus is on the development of damage evolution laws to accurately predict the degradation of strength and stiffness properties based on the load direction and the stress level. In addition to the influence of load sequence effects, particular attention will be paid to the effects of passive damage occurring under combined cyclic tension and compression loading. Finally, the extended fatigue damage model is to be applied to a fuselage structure segment of a future passenger aircraft for fatigue analysis.Led by: Prof. Dr.-Ing. habil. Raimund RolfesTeam:Year: 2020Funding: Internes ProjektDuration: seit 2020
-
Abstract modelling of the nonlinear mechanical response of joints in fiber reinforced composite assembliesIn Aviation, Automobile and Wind turbine industries, there are large composite structures which are connected using thousands of mechanical joints or adhesives. For the efficient construction of such composite structures, it is essential to evaluate the behavior of such composite joints, which is usually very complex due to the presence of non-linearities and the joint has distinct failure modes. Accurate simulation of composite joints using detailed models gives a good estimate of the joint behavior and its failure properties but it comes at the cost of computational time. The project aims to reduce the computational cost involved in the simulation of composite joints by developing an abstract model with reduced degrees of freedom. The reduction in degrees of freedom of the model is sought by using structural elements such as Shells and Beams. The project aims to create a model which will capture the behavior of the joint in the finite strain regime, such that the anisotropy of composite material and various non-linearities such as plasticity, damage, contact, friction etc. can be simulated efficiently.Led by: Prof. Dr-Ing. habil. Raimund RolfesTeam:Year: 2022Funding: DFG, German Research FoundationDuration: 01.07.2022-30.06.2025
-
Functionalized, multi-physically optimized adhesives for inherent structural health monitoring of rotor blades (Func2Ad)The performance and reliability of the rotor blade is crucial for the efficiency of a wind turbine over its entire life cycle. The blades make up a large part of the equipment cost - their manufacturing and maintenance costs are extremely high. The adhesive technology in the rotor blade is a key technology for achieving competitive advantages in the wind industry. The processing and curing properties (processability) of the adhesives as well as their operational stability (fatigue strength) in the cured state are two key parameters with regard to the system economy and the return on investment. A third would be the remote diagnosis of the glued joints of the rotor blade (Structure Health Monitoring). The research project proposed here on particle-modified adhesive systems for the wind industry starts with the three points mentioned. A main innovation is the functionalization of the adhesive resin through particle modification to implement a structural monitoring system inherent in the adhesive connections on the rotor blade. This is said to be done by modifying the electrical properties of the adhesive resin. At the same time, the processability and fatigue strength of the adhesive should be positively influenced by the modification. If the modified resin system is only optimized for one of the three aspects mentioned, there is a risk of poor performance with regard to the other. The physical properties of the adhesive must therefore not be separated for the three requirement areas, but must be considered and optimized together in their interaction and their interrelationships. In order to optimize this and increase the efficiency of the multiphysical material models, machine learning methods are used within the simulation framework.Led by: Prof. Dr-Ing. habil. Raimund RolfesTeam:Year: 2023Funding: Bundesministerium für Wirtschaft und Klimaschutz, FKZ 03EE3069 A-FDuration: 01.01.2023-31.12.2026
Structures
-
Development of a safety cockpit for gliders (CraCpit)In the joint project "CraCpit", the partners are developing solutions for a safety cockpit for gliders. The LCC and the Akaflieg München at the TU Munich are concentrating on the new development of a cockpit structure, while the ISD and the Akaflieg Hannover at the Leibniz Universität Hannover (LUH) are addressing a retrofit solution for existing aircraft types. The goal of the partners at the LUH is the design and the certifiable draft of retrofittable structural elements for the production of a safety cockpit in older, existing glider types according to current requirements. In the event of a crash, cockpit structures are subjected to high stresses that lead to large deformations and material failure. The retrofitted structural components take on different (opposing) functions, such as ensuring the pilot's survival space or energy dissipation to reduce the impact. The effectiveness of the individual components can be assessed and optimised using FEM simulations. For this purpose, appropriate material formulations are required that are able to physically represent the structural response from the onset of loading far into the post-failure range with sufficient accuracy. The focus of the work at the ISD lies in the material modelling, the simulation and the evaluation of the component function of the retrofit elements. The validation of the material models is carried out through tests at sub-component level. After the optimisation of the components through simulation, a test at the structural level (simulation of the entire fuselage and crash test of an example fuselage (prototype)) concludes the project. The experimental work is carried out in close cooperation with the partner Akaflieg Hannover e.V.. For this purpose, various test specimens and prototypes will be built and tested. In addition, an industrial partner will be involved to ensure commercial exploitation.Led by: Prof. Dr-Ing habil. Raimund RolfesTeam:Year: 2017Funding: Federal Ministry for Economic Affairs and Energy – 20E1703DDuration: 2018-2021
-
Improved structural performance through the use of random field analysisThe research performed within this project uses the effect of random variations in structure’s geometry and/or material to get information on local sensitivity of structures to deviations from their baseline value. This information cannot only be useful in quality assurance, by finding areas most sensitive to deviations, but can also be used to improve the design. This approach can load to an increase in structural parameters such as buckling load, fatigue life and others.Led by: Prof. Dr-Ing habil. Raimund RolfesTeam:Year: 2019Funding: SE²A excellence, Cluster of DFGDuration: 2019-2022
-
Multistable Morphing Structures using Variable Stiffness CompositesThe research project aims at developing multistable structures with morphing capabilities. A variable stiffness composite is used which allows stiffness tailoring with much larger design space. The developed semi analytical method is validated well within a Finite element framework. In this work, the concept of static, smart and dynamic actuations are exploited on bistable laminates to reduce the snap-through requirements.Led by: Prof. Dr-Ing habil. Raimund RolfesTeam:Year: 2019Funding: Deutscher Akademischer Austauschdienst (DAAD)Duration: 2019-2021
-
FANFOLD – Fast nonlinear machine learned analysis for rotor bladesThe performance and reliability of the rotor blade is crucial for the efficiency of a wind turbine. The blades account for a large part of the turbine costs - their repair and maintenance costs are comparatively high. Rotor blades need to be less prone to failure and less often in need of repair. Concepts of predictive maintenance and the digital twin, which will probably account for a significant part of the profits in the rotor blade market in the future, also point in this direction of reducing repair and maintenance costs. A prerequisite for the implementation of the above concepts is a fast analysis method for fibre composite structures (prediction and evaluation of damage progression and service life). For the overall simulation of the rotor blade, FE analyses using linear-elastic material models are used today. Non-linear effects due to damage or even continuous damage evolution must be investigated on a smaller scale by means of experiments or detailed simulations. In order to go one step further and, for example, to record the influence of non-linear, progressive damage processes on the aeroelasticity and service life, or in order to be able to base the quasi-static simulation in the design on less conservative reduction factors, the overall simulation on the rotor blade would have to be carried out directly taking progressive damage processes into account (load redistribution effects). Obstacles so far are the too high calculation effort and the costly experimental characterisation of existing material models. Two main topics are to be addressed in order to meet these challenges: 1. development of a new, non-linear and fast structure simulation at blade level 2. reduction of the material characterisation effort through machine learning The aim of this sub-project is a valid, efficient and cost-effective non-linear rotor blade simulation as mentioned under point one.Led by: Prof. Dr-Ing. habil. Raimund RolfesTeam:Year: 2020Funding: Federal Ministry for Economic Affairs and Energy – FKZ 03EE3028ADuration: 2020 –2023
Nanocomposites
-
Acting Principles of Nano-Scaled Matrix Additives for Composite Structures (FOR 2021)The research project aims at gaining a comprehensive understanding of the acting mechanism of nano-scaled additives to polymer matrices of continuous fibre reinforced polymer composites with respect to improved matrix dominated properties. Particularly, a sequential multi-scale simulation scheme for the prediction of mechanical properties is developed, ranging from particle-matrix interactions on nano scale up to fibre reinforced materials on micro/meso scale. It combines Finite Element and atomistic simulations based on the Molecular Dynamic Finite Element Method (MDFEM).Led by: Prof. Dr-Ing. habil. Raimund RolfesTeam:Year: 2017Funding: Deutsche Forschungsgemeinschaft (DFG)Duration: 01.07.2017 – 31.10.2020
-
Challenges of industrial application of nanomodified and hybrid material systems in lightweight rotor blade design (HANNAH)The HANNAH research project is the follow-up to the LENAH research project. In LENAH, material systems from the fields of nanomodified materials and hybrid laminates were developed, tested and numerically simulated. This allowed the high potential of these material systems for the application in rotor blade design to be demonstrated under laboratory conditions. The investigated material systems are far superior to currently established materials, especially with regard to fatigue resistance. In the follow-up project HANNAH the (further) development of production and simulation methods for these material systems for industrial standards is now in the foreground. On the one hand, the aim is to guarantee the excellent properties of the developed material systems in large-scale production and to be able to simulate the mechanical behaviour to answer industry-related issues. In this context, the ISD develops material-specific simulation models in order increase time and cost efficiency for processes of material development and component design for nanomodified materials and hybrid laminates.Led by: Prof. Dr-Ing. habil. Raimund RolfesTeam:Year: 2019Funding: Bundesministerium für Wirtschaft und Energie (BMWI) - FKZ 0324345ADuration: 01.03.2019 – 28.02.2022
-
Functionalized, multi-physically optimized adhesives for inherent structural health monitoring of rotor blades (Func2Ad)The performance and reliability of the rotor blade is crucial for the efficiency of a wind turbine over its entire life cycle. The blades make up a large part of the equipment cost - their manufacturing and maintenance costs are extremely high. The adhesive technology in the rotor blade is a key technology for achieving competitive advantages in the wind industry. The processing and curing properties (processability) of the adhesives as well as their operational stability (fatigue strength) in the cured state are two key parameters with regard to the system economy and the return on investment. A third would be the remote diagnosis of the glued joints of the rotor blade (Structure Health Monitoring). The research project proposed here on particle-modified adhesive systems for the wind industry starts with the three points mentioned. A main innovation is the functionalization of the adhesive resin through particle modification to implement a structural monitoring system inherent in the adhesive connections on the rotor blade. This is said to be done by modifying the electrical properties of the adhesive resin. At the same time, the processability and fatigue strength of the adhesive should be positively influenced by the modification. If the modified resin system is only optimized for one of the three aspects mentioned, there is a risk of poor performance with regard to the other. The physical properties of the adhesive must therefore not be separated for the three requirement areas, but must be considered and optimized together in their interaction and their interrelationships. In order to optimize this and increase the efficiency of the multiphysical material models, machine learning methods are used within the simulation framework.Led by: Prof. Dr-Ing. habil. Raimund RolfesTeam:Year: 2023Funding: Bundesministerium für Wirtschaft und Klimaschutz, FKZ 03EE3069 A-FDuration: 01.01.2023-31.12.2026
Fatigue
-
Global-local thermomechanical analysis of fracture in polycrystalline silicon shells using a phase-field approach.The photovoltaic (PV) modules containing multiple polycrystalline silicon solar cells (PSSCs) are one of the most common and widely used devices for the production of solar energy. However, the energy production efficiency degrades during their lifespan, which can be primarily associated with the cracking in a polycrystalline silicon wafer (PSW). The aim of this joint research project (ISD - Leibniz Universität Hannover, IAM - TU Braunschweig) is to evaluate the overall stiffness degradation of polycrystalline silicon solar cells (PSSCs) due to microcracking. PSSCs are intricate component consisting of multiple materials and modeling becomes computationally expensive. Therefore, modeling reduction techniques such as numerical homogenization were employed for evaluating the effective material properties of PSSCs including cracks. The cracks are to be modeled using a phase-field approach. An improved Voronoi-tessellation scheme was used to generate polycrystalline patterns of the PSW and a mean-field homogenization scheme was employed to determine the homogenized response of PSSCs. The accuracy of the homogenization scheme was verified and the material response of the heterogeneous and homogeneous PSSCs was compared.Led by: Prof. Dr-Ing. habil. Raimund RolfesTeam:Year: 2018Funding: DFG, German Research FoundationDuration: 01.08.2018 - 31.07.2021
-
Challenges of industrial application of nanomodified and hybrid material systems in lightweight rotor blade design (HANNAH)The HANNAH research project is the follow-up to the LENAH research project. In LENAH, material systems from the fields of nanomodified materials and hybrid laminates were developed, tested and numerically simulated. This allowed the high potential of these material systems for the application in rotor blade design to be demonstrated under laboratory conditions. The investigated material systems are far superior to currently established materials, especially with regard to fatigue resistance. In the follow-up project HANNAH the (further) development of production and simulation methods for these material systems for industrial standards is now in the foreground. On the one hand, the aim is to guarantee the excellent properties of the developed material systems in large-scale production and to be able to simulate the mechanical behaviour to answer industry-related issues. In this context, the ISD develops material-specific simulation models in order increase time and cost efficiency for processes of material development and component design for nanomodified materials and hybrid laminates.Led by: Prof. Dr-Ing. habil. Raimund RolfesTeam:Year: 2019Funding: Bundesministerium für Wirtschaft und Energie (BMWI) - FKZ 0324345ADuration: 01.03.2019 – 28.02.2022
-
New methods for failure and fatigue analysis of suction panels for laminar flow controlAlthough the suction panel concept holds a high potential to increase the sustainability of future aircrafts, it comes with some structural mechanical challenges that need to be carefully examined. With the panel’s underlying backbone structure adopting the load-carrying function of the outer airfoil in the suction area (see Fig. 1), the stress flux in the airfoil is considerably disturbed, resulting in multiple, potentially critical stress concentrations. To ensure a sufficient robustness of the suction panel concept in terms of static strength and fatigue resistance, the backbone structure is to be analyzed numerically by means of finite element simulations. With deep knowledge in the field of continuum damage mechanics and progressive fatigue analysis, ISD will perform high fidelity strength and fatigue analyses of the backbone structure to identify sufficiently robust designs of the backbone structure. To calibrate the numerical methods, experimental coupon tests of the backbone structure’s base material are scheduled to identify respective static and fatigue-related material properties. Beside the identification of mechanically robust designs of the suction panel, the numerical simulations are also to address topics like scalability of the suction concept and the benefits of thin ply laminates, which are well known to feature a superior fatigue resistance.Led by: Prof. Dr-Ing habil Raimund RolfesTeam:Year: 2019Funding: DFG, German Research FoundationDuration: 01.04.2019-31.12.2022
-
SE2A-Excellence Cluster sustainable and energy efficient aviationAlthough the suction panel concept holds a high potential to increase the sustainability of future aircraft, it comes with some structural mechanical challenges that need to be carefully examined. With the panel’s underlying backbone structure adopting the load-carrying function of the outer airfoil in the suction area, the stress flux in the airfoil is considerably disturbed, resulting in multiple, potentially critical stress concentrations. To ensure sufficient robustness of the suction panel concept in terms of static strength and fatigue resistance, the backbone structure is to be analyzed numerically employing finite element simulations. With deep knowledge in the field of continuum damage mechanics and progressive fatigue analysis, ISD will perform high-fidelity strength and fatigue analyses of the backbone structure to identify sufficiently robust designs of the backbone structure. From the mechanical standpoint, thin-ply (TP) laminates are known to have better static strength and fatigue resistance in contrast to conventional laminates. A well-established fatigue damage model (FDM) was calibrated and modified in order to consider the influence of ply thickness under static and cyclic loading.Led by: Prof. Dr-Ing. habil. Raimund RolfesTeam:Year: 2019Funding: DFG, German Research FoundationDuration: 01.04.2019-31.12.2022
-
Modeling and simulation of the fatigue damage behavior of fiber composites under variable block loading conditionsThe goal of this research project is the extension and application of a progressive fatigue damage model for unidirectional multi-layered fiber composites for damage analysis under variable cyclic block loading patterns. The focus is on the development of damage evolution laws to accurately predict the degradation of strength and stiffness properties based on the load direction and the stress level. In addition to the influence of load sequence effects, particular attention will be paid to the effects of passive damage occurring under combined cyclic tension and compression loading. Finally, the extended fatigue damage model is to be applied to a fuselage structure segment of a future passenger aircraft for fatigue analysis.Led by: Prof. Dr.-Ing. habil. Raimund RolfesTeam:Year: 2020Funding: Internes ProjektDuration: seit 2020
-
Experimental analysis and numerical modelling of microcrack induced delaminations under cyclic loading with load reversalsThe aim of this joint research project (ISD - Leibniz Universität Hannover, ILK - TU Dresden) is to develop a profound understanding of the damage process during delamination growth in fibre-reinforced polymer laminates (FRP) based on existing inter fibre failures during cyclic loading with load reversals. By analysing and quantifying the relevant damage processes during loading, the influence of the level and direction of the applied load on delamination growth in FRP laminates is clarified. Based on the experimental work, detailed numerical simulations on a macro- and mesoscopic level are developed which allow a purposeful analysis of the delamination process in DCB- and ENF- as well as in laminate experiments. Thus, the delamination length-dependent analysis of the fracture modes, which cannot be implemented experimentally, is made possible. Consequently, it is analysed whether characteristic values determined by standardised crack propagation investigations (DCB, ENF etc.) can be transferred to embedded layers. In addition, the investigations provide extensive experimental results on the delamination process in FRP laminates under in-plane loading and thus create a basis for the development of suitable analytical and numerical models. It is investigated to what extent existing numerical damage models (e.g. cohesive zone approaches) allow reliable and efficient modelling of cyclic delamination growth and how the mesoscopic simulation results can be used for macroscopic simulations in terms of continuum mechanics.Led by: Prof. Dr.-Ing. habil. Raimund RolfesTeam:Year: 2021Funding: German Research Foundation (DFG) - Project number 457043708Duration: 01.09.2021-31.08.2023
-
Fatigue behavior and fatigue damage prediction of short fiber - reinforced adhesives in Blades of wind turbine (Add2ReliaBlade)The reliability of rotor blades has a special importance for a safe and economic operation of wind turbines (WT). Turbine manufacturers have gained a lot of experience in the design of rotor blades and damage during operation over the past decades. Nevertheless, cracks in the rotor blade structure are still causes of costly repairs and operational failures. This indicates that there are still knowledge gaps in the design of fatigue damage in rotor blades, which is especially (but not exclusively) true for rotor blade bonding. To address these knowledge gaps and increase rotor blade reliability, the Add2RelaiBlade project was established in 2021 as a complementary addition to the ReliaBlade project. The focus of the Add2ReliaBlade project is on the development, characterization and validation of simulation methods and models for the description of the (fatigue) damage behavior of (short fiber reinforced) adhesive joints in rotor blades. The focus is on trailing edge bonding, as this is particularly susceptible to damage. This sub-project provides substantial contributions in the context of extended material testing and non-destructive imaging for short fiber reinforced adhesives, modeling of the spatial distribution of fiber orientation in short fiber reinforced trailing edge bonding, continuum mechanical and energy based modeling of the fatigue damage behavior of (short fiber reinforced) bonded joints as well as the establishment of data based methods of numerical mechanics for the analysis of fatigue damage of short fiber reinforced bonded joints.Led by: Prof. Dr.-Ing. habil. Raimund RolfesTeam:Year: 2021Funding: Federal ministry for Economic Affair and EnergyDuration: 01.05.2021-30.4.2024